Dislocations provide low-stress deformation due to the gradual sliding of atomic blocks.
Introduction
To initiate a deformation process, a certain critical resolved shear stress (CRSS) is required at the atomic level. As described in the article Fundamentals of Deformation, the theoretical values for an ideal crystal are about a factor of 1000 higher than those measured in reality.
The reason for this discrepancy are certain crystallographic defects, which occur in real metals. These are the dislocations, whereby only the edge dislocation will be discussed in this article.
Role of the dislocations in the deformation process
Due to dislocations, it is no longer necessary to break all bonds between two atomic planes at once in order to shear off a lattice planes. Rather, it is enough to overcome only one binding series at a time. The dislocation line jumps step-by-step from atomic row to atomic row with little effort and finally emerges as a slip step on the surface of the material.
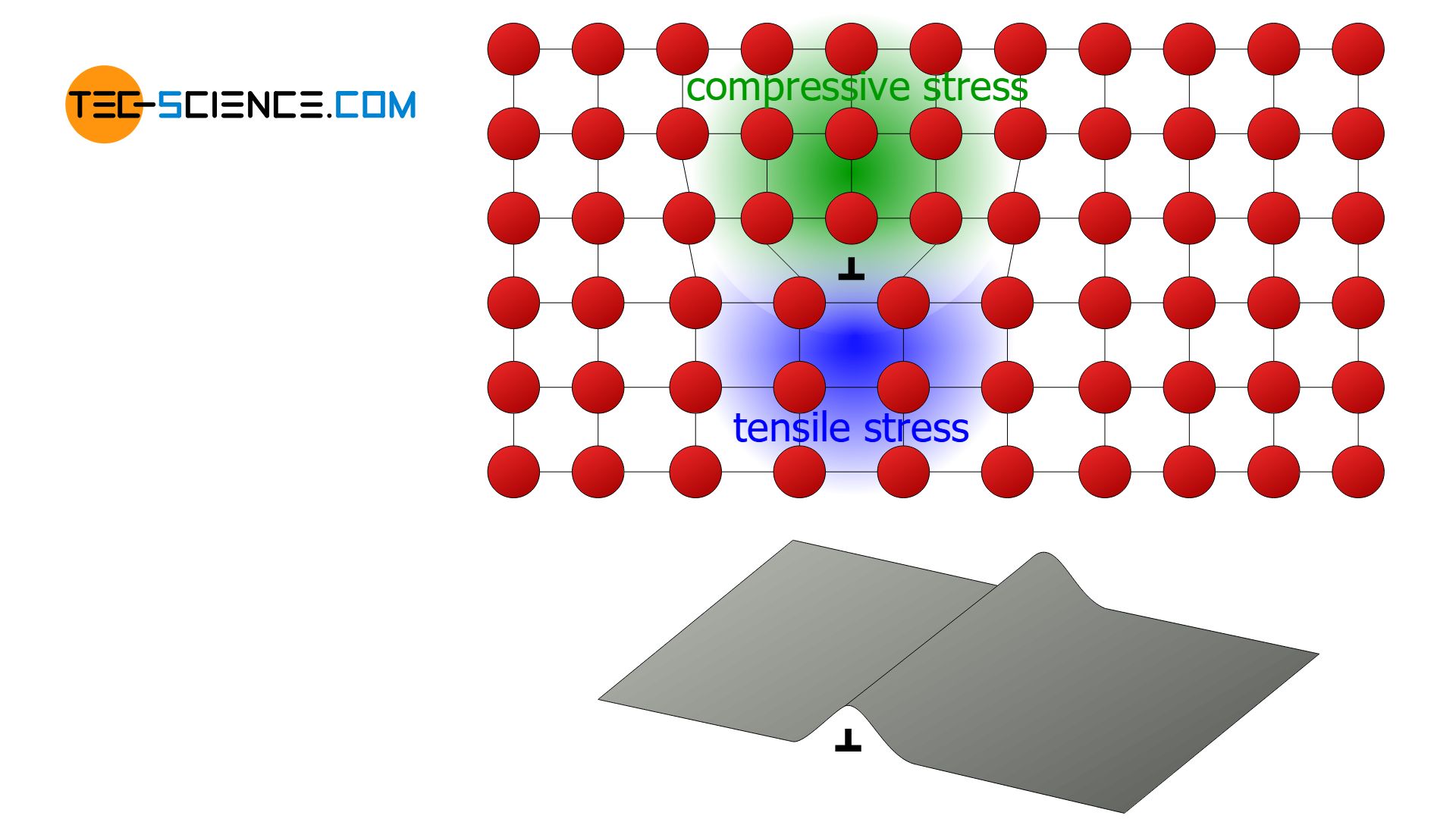
Due to the low-energy migration of the dislocations, the deformation process starts at already much lower critical shear stresses than the theory predicts without considering dislocations! This critical shear stress is referred to as Peierls stress.
Dislocations allow low-energy slippage of atomic blocks, so that deformation processes in real crystals already occur at lower critical shear stresses than in ideal crystals!
The low-energy sliding of the atomic plane by a dislocation can be illustrated by moving a carpet. Moving a large and heavy carpet usually requires a very large force due to the friction between the carpet and the floor. If, however, wrinkles are struck in the carpet and these are then moved through the carpet, then one achieves the same result with less effort. The carpet can move in stages like a caterpillar.
By understanding the atomic mechanism of deformation and the central role of dislocations, specific measures can now be taken to prevent deformation. After all, many materials that are used under high load should not deform so easily. Therefore they should be high-strength. How measures for increasing the strength of metals can look like is explained in the following section.
Strengthening mechanisms
By the term strengthening mechanisms one understands measures which aim to prevent the deformation of a metal purposefully. Thus, the highest possible strength of the corresponding material is achieved. Since the primary cause of a deformation process is the migration of dislocations, ultimately all strengthening mechanisms are based on blocking of this movement.
All measures with the aim of blocking dislocation movement are summerazied under the term strengthening mechanisms!
The most important strengthening mechanisms are described in the following sections:
- solid solution strengthening
- precipitation hardening
- grain boundary strengthening (Hall–Petch strengthening)
- work hardening (strain hardening)
Solid solution strengthening
The principle of solid solution hardening is based on the distortion of the lattice by foreign atoms. These can be either substitutional atoms or interstitial atoms. Due to their blocking of the dislocation movement, the lattice planes can consequently no longer slide off so easily. A deformation of the lattice thus occurs only at significantly higher critical shear stresses, since the lattice distortion must also be overcome. In this way, an increase in the strength of the material is ultimately achieved.
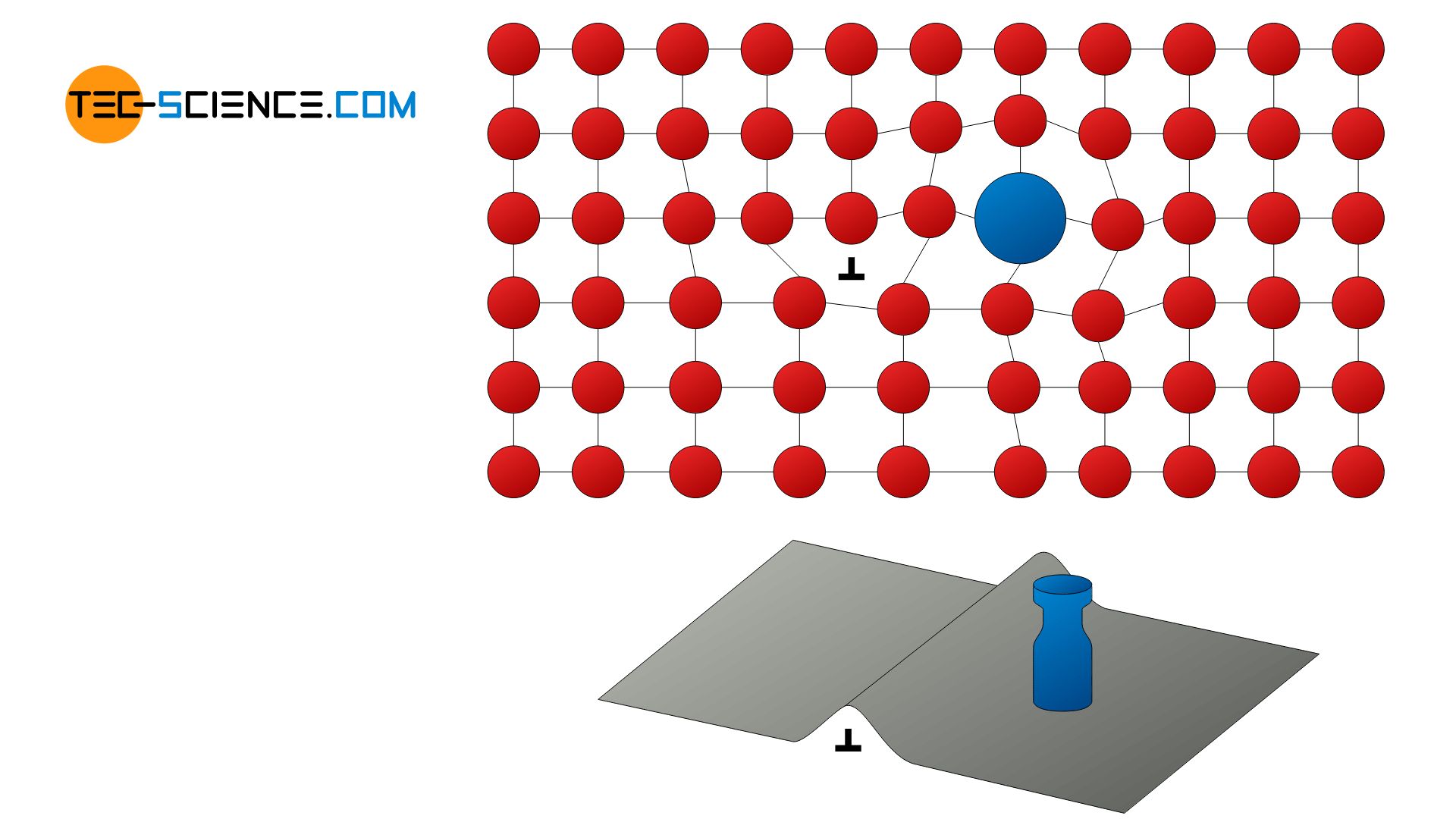
With solid solution hardening, foreign atoms block the dislocation movement!
Precipitation strengthening
Not only single foreign atoms can impede the dislocation movement, but precipitates can also block the movement of dislocations. This is called precipitation hardening or precipitation strengthening.
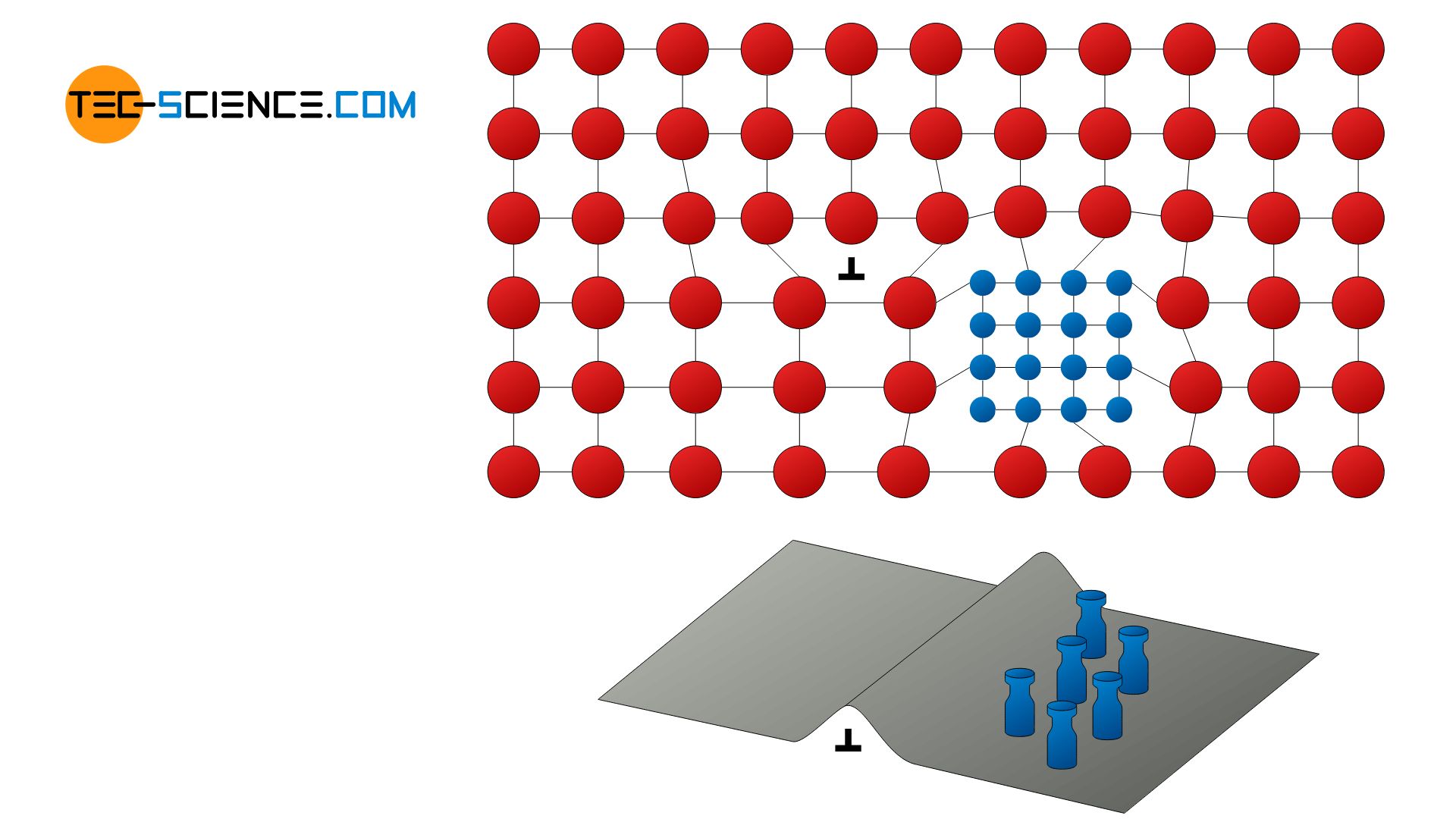
In a precipitation hardening precipitates block the dislocation movement!
This principle of precipitation strengthening is used in so-called hardenable aluminum alloys. The aluminum alloy is first heated to a relatively high temperature, so that the foreign atoms contained therein can completely dissolve in the aluminum lattice structure. Note that solubility generally decreases with decreasing temperature, so high temperatures are required for complete solubility.
If it is cooled rapidly (called quenching), then the foreign atoms, despite the lower solubility, remain forcibly dissolved in the lattice. Since the concentration of dissolved atoms in this state is above the actual solubility limit, one speaks of a so-called supersaturated solid solution.
This state is not thermodynamically stable, so that the forcibly solved foreign atoms begin to segregate from the lattice and form their own compounds (precipitates) within the metal. To accelerate this process of so-called aging, the alloy is heated slightly, so that the diffusion processes can proceed more quickly.
Grain boundary hardening (grain refining)
The principle of grain boundary hardening is based on the complicated dislocation movement across grain boundaries. Grain boundaries are therefore no weak points in the material in this context but contribute to a particular extent to the increase in strength!
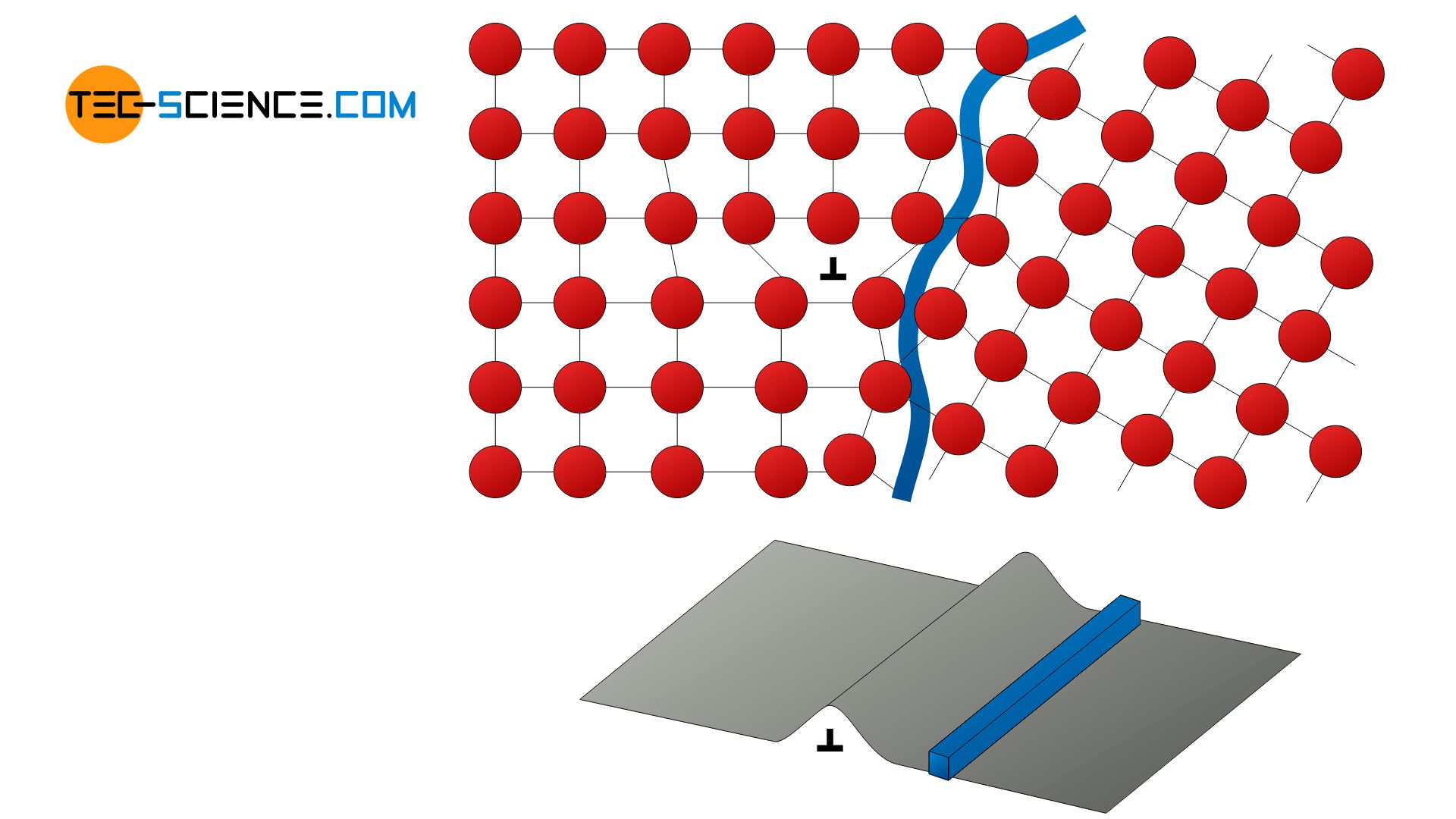
A high number of grain boundaries can be achieved by fine grains in the material. Therefore, grain boundary strengthening is also called grain refinement (sometimes referred to as Hall–Petch strengthening). A small grain size can be achieved by targeted influencing of the melt during cooling (for example by seeding or supercooling of the melt).
With a grain refining, grain boundaries block the dislocation movement!
The principle of grain refining is applied to so-called weldable fine grain steels in steel building. Although carbon has a strength-increasing effect in steel, it is undesirable in terms of good weldability. Carbon makes the steel hard and brittle due to the rapid cooling after welding. Therefore, due to a good weldability (as is often required in steel building), it is necessary to keep the carbon content as low as possible. Nevertheless, in order to ensure a high strength, one is dependent on grain refining. The diameters of the individual grains in fine grain steels are in the range of approximately 20 μm.
Work hardening (strain strengthening)
The principle of work hardening is based on the introduction of additional dislocations during plastic deformation. In every deformation process, new dislocations are always introduced into the material. The dislocations thus hinder each other from moving, which results in a strength-increasing effect.
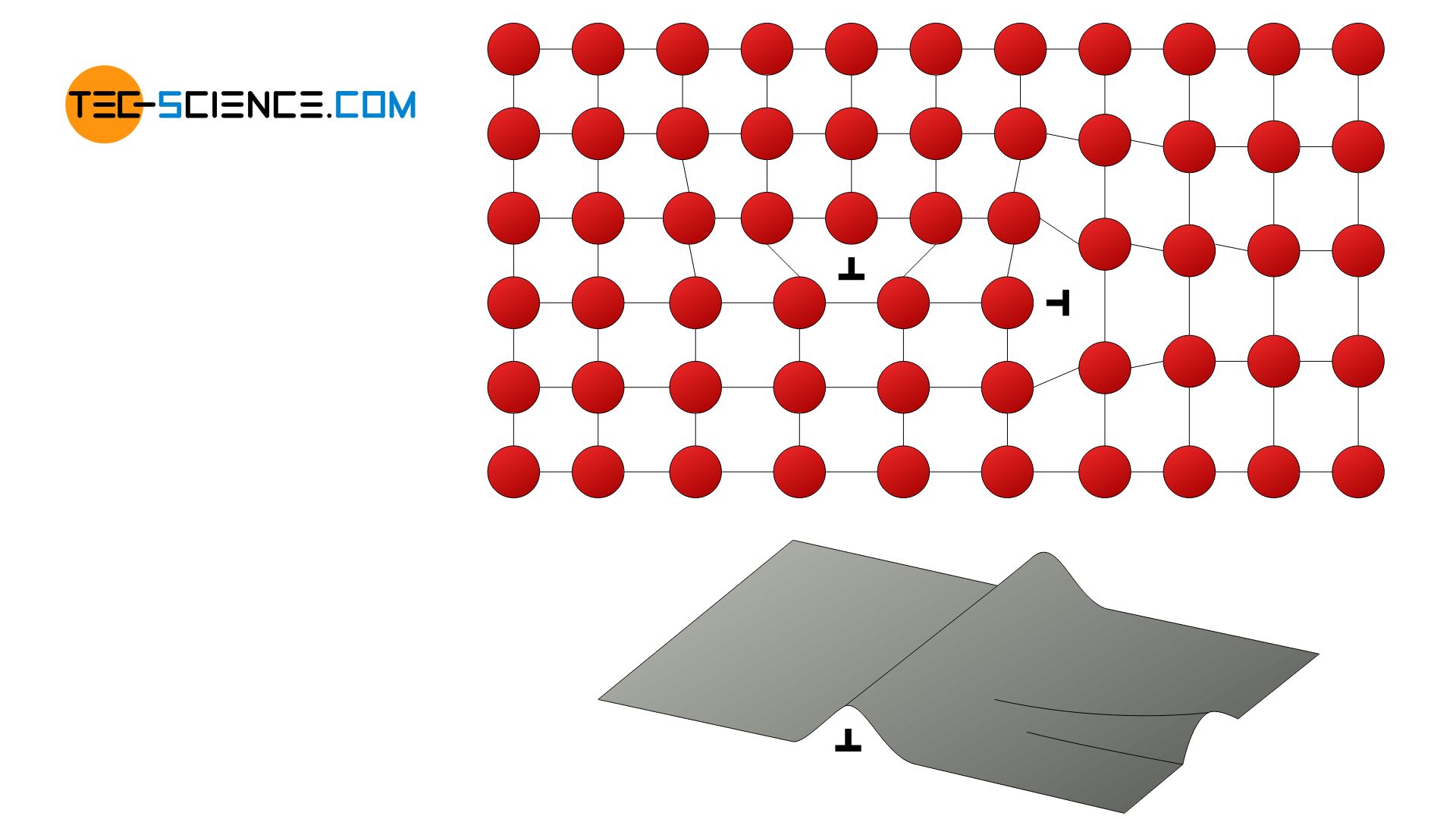
With work hardening additionally introduced dislocations block each other from moving!
The increase in strength during plastic deformation can also be comprehended using the stress-strain diagram. For this purpose, a tensile specimen is first stretched out beyond the yield strength \(R_p\) (red line to point A) and therefore suffers a plastic deformation.
If the force is subsequently removed, the operating point returns to zero stress in a direction parallel to Hooke’s straight line (point B). Accordingly, the material has a permanent elongation.
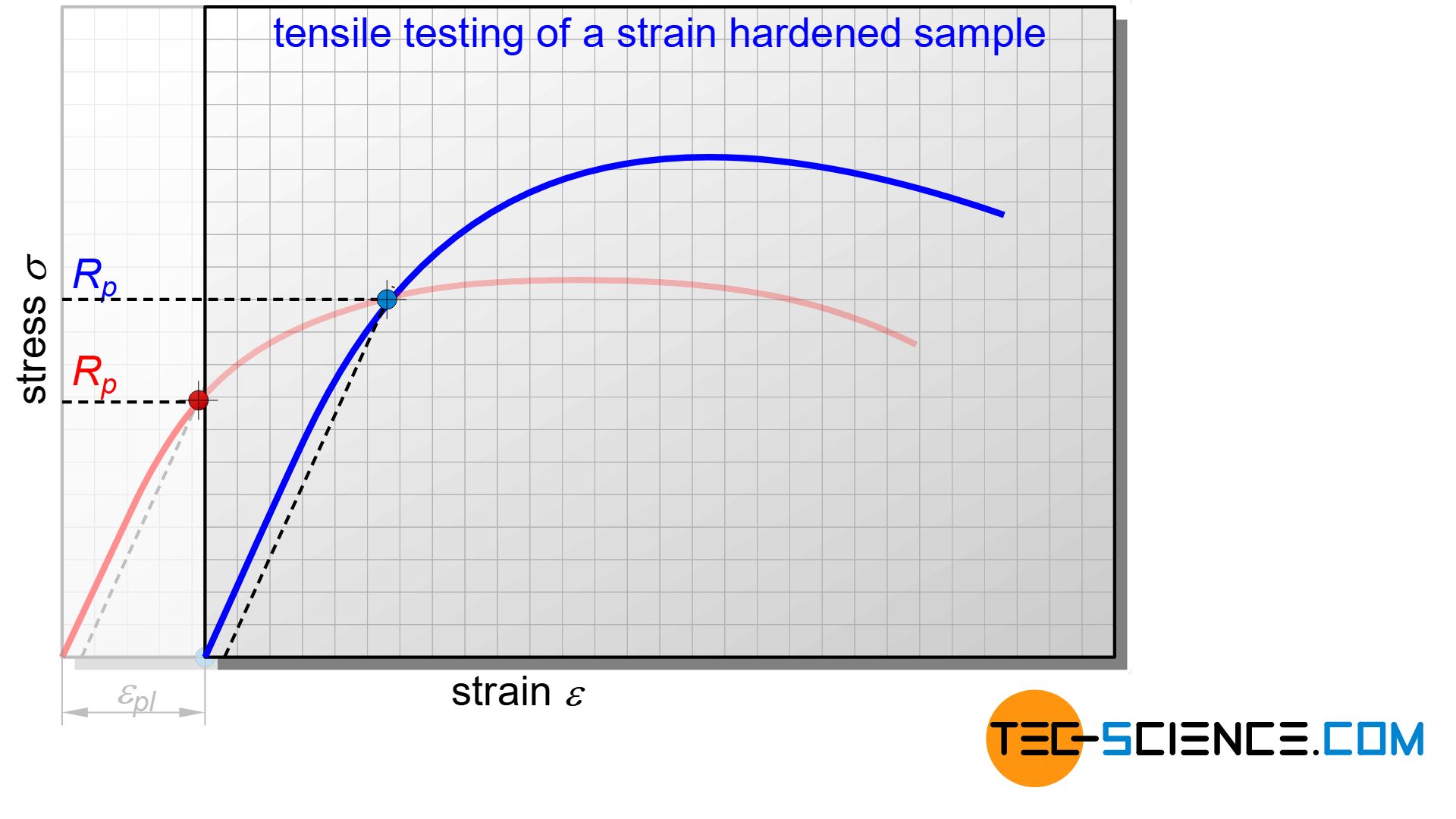
If the tensile test is repeated again, the operating point first goes up the elastic straight line again and only reaches plastic deformation at higher stress (blue curve)!
The comparison of both stress–strain curves makes it clear that the plastic deformation now only occurs at higher stress. This means that the work-hardened sample obviously has an increased yield strength \(R_p\)! The tensile strength also increases accordingly. Due to the strain a workpiece has suffered during plastic deformation, work hardening is also referred to as strain strengthening.
Work hardening is specifically brought about, for example, in the manufacture of cold-rolled sheets in order to achieve a significantly higher strength compared to the hot-rolled condition.
Note that work hardening can not be done to any degree. If too many dislocations are introduced by plastic deformation, the material is thereby locally destroyed and ruptured.
This behavior is evident, for example, in the repeated bending back and forth of a wire. This only works well until too many dislocations have been introduced and the wire eventually breaks.